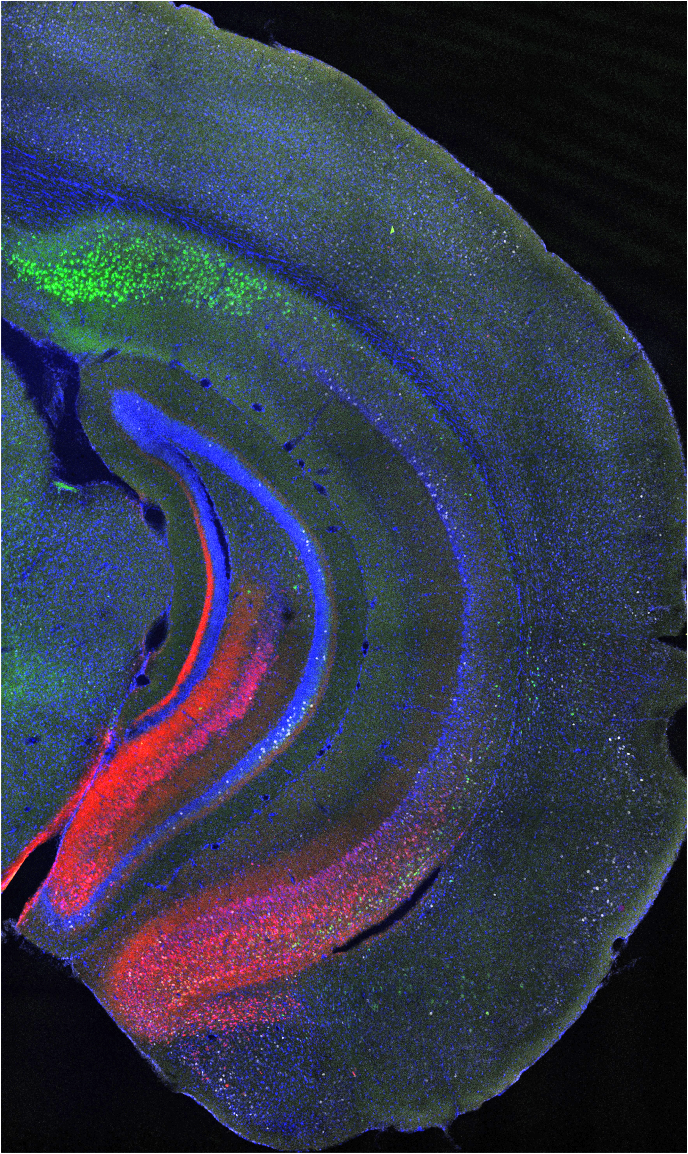
Research
The Kheirbek Lab's research goal is to delineate the neuronal circuits that underlie emotional behavior, and understand how they go awry in psychiatric illnesses— such as mood and anxiety disorders.
We study how emotionally-relevant stimuli are encoded within neural networks and how these representations change with learning. We use an integrative approach that leverages state-of-the-art technologies to monitor and control genetically-identified brain networks in real time.
Research Projects
Ventral hippocampal control of emotional behavior
Almost a third of adult Americans suffer from an anxiety disorder, carrying an enormous personal, societal, and financial burden. Anxiety disorders are complex circuit-based conditions, resulting from dysfunction in several distributed regions, connections, and cell types that generate normal adaptive behavior. Thus, a deeper understanding of how these neural circuits become disrupted to generate maladaptive behavior is crucial if we hope to generate new treatments.
In recent years, we have shown that the ventral hippocampus is a central node in the extended circuit that generates adaptive avoidance behavior. In this work we aim to understand the input- output organization of the ventral hippocampus in the control of mood and anxiety-related behavior. We use functional imaging of large populations of anatomically defined vHPC neurons, optical manipulation of these neurons, and high-resolution behavioral analyses to expand our understanding of how the extended ventral hippocampal network controls behavior. It is our goal to provide a detailed functional map of the extended hippocampal circuit that controls avoidance behavior, and provide novel targets for generation of therapeutics for the treatment of anxiety disorders.
Neural dynamics in the hippocampus that support associative learning
The brain transforms experiences into patterns of activity that control emotions, decisions and behaviors. Discriminating these patterns of activity allow these experiences to be stored as distinct entities, separating important stimuli from unimportant ones, and storing them into memory. A locus of this computation is the hippocampus, which, with learning, encodes the relationships and distinctions between behaviorally relevant variables.
We have recently found that the dentate gyrus subregion of the hippocampus classifies cortical representations of olfactory stimuli, increasing the distance between odor representations with learning. In addition, we found that ventral CA1 region of the hippocampus only discriminates incoming sensory information when behavioral significance has been attached to the stimulus. In this work, we aim to understand the mechanisms that support learning-induced flexibility of neural ensembles in the hippocampus.
New myelin in the long-term storage of fear memories
Active myelination of adult brain circuits has been shown to be important for some forms of learning, and recent work from our groups and others has shown that this a crucial process in memory storage and retrieval. Myelination increases conduction velocity across individual axons, however how this translates to computations at the level of neural circuits and their subsequent behavioral outputs is poorly understood. In this work, we aim to understand how this process of active myelination in the adult brain modulates the dynamic nature of neural encoding within memory circuits.
We aim to understand the cell types in the mouse cortex that become myelinated after a learning experience, how active myelination of cortical circuits impacts the cellular codes that support memory, how activity-dependent myelination modulates the synchronization and interregional communication between brain areas, and the temporal dynamics of oligodendrocyte precursor proliferation and differentiation in vivo after memory encoding. It is our goal to understand fundamental mechanisms of glial signaling during learning and memory. This work is in close collaboration with Jonah Chan’s lab at UCSF.
Brain-wide neural signatures for mood and anxiety
The underlying circuits that are disrupted in mood and anxiety disorders are incredibly complex. To understand them, an integrative approach is required that combines animal models with state-of-the-art tools to dissect the circuits that are disrupted to produce maladaptive behavior. In this line of work, we use multi-region, high-density electrophysiology to identify biomarkers for changes in mood related behavior and for stratifying susceptibility and resiliency to chronic stress. We also ask how therapeutics may modify these biomarkers and behavior, with a recent interest in psychedelics such as psilocybin.
In addition, we study the impact of bodily signals in the generation of behavior, and how these interoceptive signals are encoded in the brain. Using genetic and anatomical tools, we dissect the structure of these relevant circuits and use optical tools to control their activity to identify novel targets for neuromodulation.
Tools
We use a combination of state-of-the-art tools to dissect the neural circuits that give rise to emotional behavior.
2-photon calcium imaging
Behavior
In vivo electrophysiology
1-photon freely moving calcium imaging
Fiber photometry
Anatomy
Ex vivo electrophysiology
Optogenetics